Part 4: Pediatric Basic and Advanced Life Support
- High-quality cardiopulmonary resuscitation (CPR) is the foundation of resuscitation. New data reaffirm the key components of high-quality CPR: providing adequate chest compression rate and depth, minimizing interruptions in CPR, allowing full chest recoil between compressions, and avoiding excessive ventilation.
- A respiratory rate of 20 to 30 breaths per minute is new for infants and children who are (a) receiving CPR with an advanced airway in place or (b) receiving rescue breathing and have a pulse.
- For patients with nonshockable rhythms, the earlier epinephrine is administered after CPR initiation, the more likely the patient is to survive.
- Using a cuffed endotracheal tube decreases the need for endotracheal tube changes.
- The routine use of cricoid pressure does not reduce the risk of regurgitation during bag-mask ventilation and may impede intubation success.
- For out-of-hospital cardiac arrest, bag-mask ventilation results in the same resuscitation outcomes as advanced airway interventions such as endotracheal intubation.
- Resuscitation does not end with return of spontaneous circulation (ROSC). Excellent post–cardiac arrest care is critically important to achieving the best patient outcomes. For children who do not regain consciousness after ROSC, this care includes targeted temperature management and continuous electroencephalography monitoring. The prevention and/or treatment of hypotension, hyperoxia or hypoxia, and hypercapnia or hypocapnia is important.
- After discharge from the hospital, cardiac arrest survivors can have physical, cognitive, and emotional challenges and may need ongoing therapies and interventions.
- Naloxone can reverse respiratory arrest due to opioid overdose, but there is no evidence that it benefits patients in cardiac arrest.
- Fluid resuscitation in sepsis is based on patient response and requires frequent reassessment. Balanced crystalloid, unbalanced crystalloid, and colloid fluids are all acceptable for sepsis resuscitation. Epinephrine or norepinephrine infusions are used for fluid-refractory septic shock.
More than 20 000 infants and children have a cardiac arrest per year in the United States.1–4 In 2015, emergency medical service–documented out-of-hospital cardiac arrest (OHCA) occurred in more than 7000 infants and children.4 Approximately 11.4% of pediatric OHCA patients survived to hospital discharge, but outcomes varied by age, with survival rates of 17.1% in adolescents, 13.2% in children, and 4.9% in infants. In the same year, pediatric in-hospital cardiac arrest (IHCA) incidence was 12.66 events per 1000 infant and child hospital admissions, with an overall survival to hospital discharge rate of 41.1%.4 Neurological outcomes remain difficult to assess across the pediatric age spectrum, with variability in reporting metrics and time to follow-up across studies of both OHCA and IHCA. Favorable neurological outcome has been reported in up to 47% of survivors to discharge.5 Despite increases in survival from IHCA, there is more to be done to improve both survival and neurological outcomes.6
The International Liaison Committee on Resuscitation (ILCOR) Formula for Survival emphasizes 3 essential components for good resuscitation outcomes: guidelines based on sound resuscitation science, effective education of the lay public and resuscitation providers, and implementation of a well-functioning Chain of Survival.7
These guidelines contain recommendations for pediatric basic and advanced life support, excluding the newborn period, and are based on the best available resuscitation science. The Chain of Survival (Section 2), which is now expanded to include recovery from cardiac arrest, requires coordinated efforts from medical professionals in a variety of disciplines and, in the case of OHCA, from bystanders, emergency dispatchers, and first responders. In addition, specific recommendations about the training of resuscitation providers are provided in Part 6: Resuscitation Education Science, and recommendations about systems of care are provided in Part 7.
These guidelines are intended to be a resource for lay rescuers and healthcare providers to identify and treat infants and children in the prearrest, intra-arrest, and postarrest states. These apply to infants and children in multiple settings; the community, prehospital, and the hospital environment. Prearrest, intra-arrest, and postarrest topics are reviewed, including cardiac arrest in special circumstances, such as in patients with congenital heart disease.
For the purposes of the pediatric advanced life support guidelines, pediatric patients are infants, children, and adolescents up to 18 years of age, excluding newborns. For pediatric basic life support (BLS), guidelines apply as follows:
- Infant guidelines apply to infants younger than approximately 1 year of age.
- Child guidelines apply to children approximately 1 year of age until puberty. For teaching purposes, puberty is defined as breast development in females and the presence of axillary hair in males.
- For those with signs of puberty and beyond, adult basic life support guidelines should be followed.
Resuscitation of the neonate is addressed in “Part 5: Neonatal Resuscitation” and applies to the newborn typically only during the first hospitalization following birth. Pediatric basic and advanced life support guidelines apply to neonates (less than 30 days old) after hospital discharge.
COVID-19 Guidance
Together with other professional societies, the AHA has provided interim guidance for basic and advanced life support in adults, children, and neonates with suspected or confirmed coronavirus disease 2019 (COVID-19). Because evidence and guidance are evolving with the COVID-19 situation, this interim guidance is maintained separately from the emergency cardiovascular care (ECC) guidelines. Readers are directed to the American Heart Association (AHA) website for the most recent guidance.8
The Pediatric Writing Group consisted of pediatric clinicians including intensivists, cardiac intensivists, cardiologists, emergency medicine physicians, medical toxicologists, and nurses. Volunteers with recognized expertise in resuscitation are nominated by the writing group chair and selected by the AHA ECC Committee. The AHA has rigorous conflict of interest policies and procedures to minimize the risk of bias or improper influence during development of the guidelines.9 Prior to appointment, writing group members and peer reviewers disclosed all commercial relationships and other potential (including intellectual) conflicts. Writing group members whose research led to changes in guidelines were required to declare those conflicts during discussions and abstain from voting on those specific recommendations. This process is described more fully in “Part 2: Evidence Evaluation and Guidelines Development.” Disclosure information for writing group members is listed in Appendix 1.
These pediatric guidelines are based on the extensive evidence evaluation performed in conjunction with the ILCOR and affiliated ILCOR member councils. Three different types of evidence reviews (systematic reviews, scoping reviews, and evidence updates) were used in the 2020 process.10,11 After review by the ILCOR Science Advisory Committee Chair, the evidence update worksheets were included in Appendix C of the 2020 ILCOR Consensus on CPR and ECC Science With Treatment Recommendations.11a Each of these resulted in a description of the literature that facilitated guideline development. This process is described more fully in “Part 2: Evidence Evaluation and Guidelines Development.”12
The writing group reviewed all relevant and current AHA Guidelines for Cardiopulmonary Resuscitation (CPR) and ECC and all relevant 2020 ILCOR Consensus on CPR and ECC Science With Treatment Recommendations evidence and recommendations to determine if current guidelines should be reaffirmed, revised, or retired or if new recommendations were needed. The writing group then drafted, reviewed, and approved recommendations, assigning to each a Class of Recommendation (COR; ie, strength) and Level of Evidence (LOE; ie, quality, certainty). Criteria for each COR and LOE are described in Table 1.
Open table in a new window
The 2020 Guidelines are organized in discrete modules of information on specific topics or management issues.13 Each modular “knowledge chunk” includes a table of recommendations using standard AHA nomenclature of COR and LOE. Recommendations are presented in order of COR: most potential benefit (Class 1), followed by lesser certainty of benefit (Class 2), and finally potential for harm or no benefit (Class 3). Following the COR, recommendations are ordered by the certainty of supporting LOE: Level A (high-quality randomized controlled trials) to Level C-EO (expert opinion). This order does not reflect the order in which care should be provided.
A brief introduction or short synopsis is provided to contextualize the recommendations with important background information and overarching management or treatment concepts. Recommendation-specific supportive text clarifies the rationale and key study data supporting the recommendations. When appropriate, flow diagrams or additional tables are included. Hyperlinked references are provided to facilitate quick access and review.
The guideline was submitted for blinded peer review to 5 subject matter experts nominated by the AHA. Peer reviewer feedback was provided for guidelines in draft format and again in final format. The guideline was also reviewed and approved for publication by the AHA Science Advisory and Coordinating Committee and AHA Executive Committee. Disclosure information for peer reviewers is listed in Appendix 2.
Abbreviation | Meaning/Phrase |
---|---|
ACLS | advanced cardiovascular life support |
AED | automated external defibrillator |
ALS | advanced life support |
AHA | American Heart Association |
BLS | basic life support |
COI | conflict of interest |
COR | Class of Recommendation |
CPR | cardiopulmonary resuscitation |
ECC | emergency cardiovascular care |
ECLS | extracorporeal life support |
ECMO | extracorporeal membrane oxygenation |
ECPR | extracorporeal cardiopulmonary resuscitation |
EO | Expert Opinion |
ETI | endotracheal intubation |
FBAO | foreign body airway obstruction |
IHCA | in-hospital cardiac arrest |
ILCOR | International Liaison Committee on Resuscitation |
LD | limited data |
LOE | Level of Evidence |
MCS | mechanical circulatory support |
NR | nonrandomized |
OHCA | out-of-hospital cardiac arrest |
PALS | pediatric advanced life support |
PICO | population, intervention, comparator, outcome |
pVT | pulseless ventricular tachycardia |
RCT | randomized clinical trial |
ROSC | return of spontaneous circulation |
SGA | supraglottic airway |
TTM | targeted temperature management |
VF | ventricular fibrillation |
The epidemiology, pathophysiology, and common etiologies of pediatric cardiac arrest are distinct from adult and neonatal cardiac arrest. Cardiac arrest in infants and children does not usually result from a primary cardiac cause; rather, it is the end result of progressive respiratory failure or shock. In these patients, cardiac arrest is preceded by a variable period of deterioration, which eventually results in cardiopulmonary failure, bradycardia, and cardiac arrest. In children with congenital heart disease, cardiac arrest is often due to a primary cardiac cause, although the etiology is distinct from adults.
Outcomes for pediatric IHCA have improved over the past 20 years, in part because of early recognition, high-quality CPR, postarrest care, and extracorporeal cardiopulmonary resuscitation (ECPR).1,2 In a recent analysis of the Get With The Guidelines Resuscitation Registry, a large multicenter, hospital-based cardiac arrest registry, pediatric cardiac arrest survival to hospital discharge was 19% in 2000 and 38% in 2018.2 Survival has increased on average by 0.67% per year, though that increase has plateaued since 2010.2 New directions of research and therapy may be required to improve cardiac arrest survival. More cardiac arrest events now occur in an intensive care unit (ICU) setting, suggesting that patients at risk for cardiac arrest are being identified sooner and transferred to a higher level of care.3
Survival rates from OHCA remain less encouraging. In a recent analysis of the Resuscitation Outcomes Consortium Epidemiological Registry, a multicenter OHCA registry, annual survival to hospital discharge of pediatric OHCA between 2007 and 2012 ranged from 6.7% to 10.2% depending on region and patient age.4 There was no significant change in these rates over time, consistent with other national registries from Japan and from Australia and New Zealand.5,6 In the Resuscitation Outcomes Consortium Epidemiological Registry, survival of OHCA was higher in regions with more arrests that were witnessed by emergency medical services and with higher bystander CPR rates, stressing the importance of early recognition and treatment of these patients.4
As survival rates from pediatric cardiac arrest increase, there has been a shift with more focus on neurodevelopmental, physical, and emotional outcomes of survivors. Recent studies demonstrate that a quarter of patients with favorable outcomes have global cognitive impairment and that 85% of older children who were reported to have favorable outcomes have selective neuropsychological deficits.7
Historically, cardiac arrest care has largely focused on the management of the cardiac arrest itself, highlighting high-quality CPR, early defibrillation, and effective teamwork. However, there are aspects of prearrest and postarrest care that are critical to improve outcomes. As pediatric cardiac arrest survival rates have plateaued, the prevention of cardiac arrest becomes even more important. In the out-of-hospital environment, this includes safety initiatives (eg, bike helmet laws), sudden infant death syndrome prevention, lay rescuer CPR training, and early access to emergency care. When OHCA occurs, early bystander CPR is critical in improving outcomes. In the in-hospital environment, cardiac arrest prevention includes early recognition and treatment of patients at risk for cardiac arrest such as neonates undergoing cardiac surgical procedures, patients presenting with acute fulminant myocarditis, acute decompensated heart failure, or pulmonary hypertension.
Following resuscitation from cardiac arrest, management of the post–cardiac arrest syndrome (which may include brain dysfunction, myocardial dysfunction with low cardiac output, and ischemia or reperfusion injury) is important to avoid known contributors to secondary injury, such as hypotension.8,9 Accurate neuroprognostication is important to guide caregiver discussions and decision-making. Finally, given the high risk of neurodevelopmental impairment in cardiac arrest survivors, early referral for rehabilitation assessment and intervention is key.
To highlight these different aspects of cardiac arrest management, the Pediatric Chain of Survival has been updated (Figure 1). A separate OHCA Chain of Survival has been created to distinguish the differences between OHCA and IHCA. In both the OHCA and IHCA chains, a sixth link has been added to stress the importance of recovery, which focuses on short- and long-term treatment evaluation, and support for survivors and their families. For both chains of survival, activating the emergency response is followed immediately by the initiation of high-quality CPR. If help is nearby or a cell phone is available, activating the emergency response and starting CPR can be nearly simultaneous. However, in the out-of-hospital setting, a single rescuer who does not have access to a cell phone should begin CPR (compressions-airway-breathing) for infants and children before calling for help because respiratory arrest is the most common cause of cardiac arrest and help may not be nearby. In the event of sudden witnessed collapse, rescuers should use an available automatic external defibrillator (AED), because early defibrillation can be lifesaving.
Rapid recognition of cardiac arrest, immediate initiation of high-quality chest compressions, and delivery of effective ventilations are critical to improve outcomes from cardiac arrest. Lay rescuers should not delay starting CPR in a child with no “signs of life.” Healthcare providers may consider assessing the presence of a pulse as long as the initiation of CPR is not delayed more than 10 seconds. Palpation for the presence or absence of a pulse is not reliable as the sole determinant of cardiac arrest and the need for chest compressions. In infants and children, asphyxial cardiac arrest is more common than cardiac arrest from a primary cardiac event; therefore, effective ventilation is important during resuscitation of children. When CPR is initiated, the sequence is compressions-airway-breathing.
High-quality CPR generates blood flow to vital organs and increases the likelihood of return of spontaneous circulation (ROSC). The 5 main components of high-quality CPR are (1) adequate chest compression depth, (2) optimal chest compression rate, (3) minimizing interruptions in CPR (ie, maximizing chest compression fraction or the proportion of time that chest compressions are provided for cardiac arrest), (4) allowing full chest recoil between compressions, and (5) avoiding excessive ventilation. Compressions of inadequate depth and rate,1,2 incomplete chest recoil,3 and high ventilation rates4,5 are common during pediatric resuscitation.
COR | LOE | Recommendations |
---|---|---|
1 | C-LD |
|
2a | C-LD |
|
2b | C-EO |
|
Recommendation-Specific Supportive Text
- Lay rescuers are unable to reliably determine the presence or absence of a pulse.6–20
- No clinical trials have compared manual pulse checks with observations of “signs of life.” However, adult and pediatric studies have identified a high error rate and harmful CPR pauses during manual pulse checks by trained rescuers.21–23 In 1 study, healthcare provider pulse palpation accuracy was 78%21 compared with lay rescuer pulse palpation accuracy of 47% at 5 seconds and 73% at 10 seconds.6
- One pediatric study demonstrated only a small delay (5.74 seconds) in commencement of rescue breathing with compressions-airway-breathing compared with airway-breathing-compressions.24 Although the evidence is of low certainty, continuing to recommend compressions-airway-breathing likely results in minimal delays in rescue breathing and allows for a consistent approach to cardiac arrest treatment in adults and children.
COR | LOE | Recommendations |
---|---|---|
1 | B-NR |
|
1 | B-NR | |
1 | C-EO | |
2a | C-LD | |
2a | C-LD |
|
2a | C-EO |
|
2a | C-EO |
|
2a | C-EO |
|
2b | C-LD |
|
Recommendation-Specific Supportive Text
- Large observational studies of children with OHCA show the best outcomes with compression-ventilation CPR, though outcomes for infants with OHCA are often poor regardless of resuscitation strategy.25–29
- Large observational studies of children with OHCA show that compression-only CPR is superior to no bystander CPR, though outcomes for infants with OHCA are often poor.27,28
- Allowing complete chest re-expansion improves the flow of blood returning to the heart and thereby blood flow to the body during CPR. There are no pediatric studies evaluating the effect of residual leaning during CPR, although leaning during pediatric CPR is common.2,3 In 1 observational study of invasively monitored and anesthetized children, leaning was associated with elevated cardiac filling pressures, leading to decreased coronary perfusion pressures during sinus rhythm.30
- A small observational study found that a compression rate of at least 100/min was associated with improved systolic and diastolic blood pressures during CPR for pediatric IHCA.31 One multicenter, observational study of pediatric IHCA demonstrated increased systolic blood pressures with chest compression rates between 100 and 120/min when compared with rates exceeding 120/min.32 Rates less than 100/min were associated with improved survival compared to rates of 100 to 120/min; however, the median rate in this slower category was approximately 95/min (ie, very close to 100/min).32
- Three anthropometric studies have shown that the pediatric chest can be compressed to one third of the anterior-posterior chest diameter without damaging intrathoracic organs.33–35 An observational study found an improvement in rates of ROSC and 24-hour survival, when at least 60% of 30-second epochs of CPR achieve an average chest compression depth greater than 5 cm for pediatric IHCA.36
- Current recommendations include a brief rhythm check every 2 minutes when a monitor or AED is available.
- There are no human studies addressing the effect of varying inhaled oxygen concentrations during CPR on outcomes in infants and children.
- The optimum compression-to-ventilation ratio is uncertain. Large observational studies of children with OHCA demonstrated better outcomes with compression-ventilation CPR with ratios of either 15:2 or 30:2 compared with compression-only CPR.25
- One small, multicenter observational study of intubated pediatric patients found that ventilation rates (at least 30 breaths/min in children less than 1 year of age, at least 25 breaths/min in older children) were associated with improved rates of ROSC and survival.5 However, increasing ventilation rates are associated with decreased systolic blood pressure in children. The optimum ventilation rate during continuous chest compressions in children with an advanced airway is based on limited data and requires further study.
Recommendations 1 and 2 were reviewed in the “2017 American Heart Association Focused Update on Pediatric Basic Life Support and Cardiopulmonary Resuscitation Quality: An Update to the American Heart Association Guidelines for Cardiopulmonary Resuscitation and Emergency Cardiovascular Care.”37
COR | LOE | Recommendations |
---|---|---|
1 | C-LD |
|
1 | C-LD |
|
2b | C-LD |
|
2b | C-EO |
|
Recommendation-Specific Supportive Text
- One anthropometric38 and 3 radiological studies39–41 found that optimal cardiac compressions occur when fingers are placed just below the intermammary line. One observational pediatric study found that blood pressure was higher when compressions were performed over the lower third of the sternum compared to the midsternum.41 See Figure 2 for the 2-finger technique.
- Systematic reviews suggest that the 2-thumb– encircling hands technique may improve CPR quality when compared with 2-finger compressions, particularly for depth.42,43 However, recent manikin studies suggest that the 2-thumb–encircling hands technique may be associated with lower chest compression fractions (percent of cardiac arrest time that chest compression are provided)44 and incomplete chest recoil,45,46 especially when performed by single rescuers. See Figure 3 for the 2-thumb–encircling hands technique.
- There are no pediatric-specific clinical data to determine if the 1-hand or 2-hand technique produces better outcomes for children receiving CPR. In manikin studies, the 2-hand technique has been associated with improved compression depth,47 compression force,48 and less rescuer fatigue.49
- There were no human studies comparing the 1-hand compression versus the 2-thumb–encircling hands technique in infants.
Recommendation-Specific Supportive Text
- “CPR mode” is available on some hospital beds to stiffen the mattress during CPR. Manikin models indicate that mattress compression ranges between 12% and 57% of total compression depth, with softer mattresses being compressed the most.50–53 This can lead to reduced sternal displacement and a reduction in effective chest compression depth.
- Manikin studies and 1 pediatric case series show that effective compression depth can be achieved even on a soft surface, providing the CPR provider increases overall compression depth to compensate for mattress compression.53–59
- Meta-analysis of 6 studies53,56,60–63 showed a 3-mm (95% CI 1–4 mm) improvement in chest compression depth associated with backboard use when CPR was performed on a manikin placed on a mattress or bed.
COR | LOE | Recommendations |
---|---|---|
1 | C-LD |
|
1 | C-EO |
|
1 | C-EO |
|
Recommendation-Specific Supportive Text
- No data directly address the ideal method to open or maintain airway patency. One retrospective cohort study evaluated various head-tilt angles in neonates and young infants undergoing diagnostic MRI and found that the highest proportion of patent airways was at a head-tilt angle of 144 to 150 degrees based on a regression analysis.64
- While no pediatric studies evaluate jaw thrust versus head tilt–chin lift to open the airway, the jaw thrust is widely accepted as an effective way to open the airway, and this maneuver theoretically limits cervical motion compared with the head tilt–chin lift.
- There are no pediatric studies evaluating the impact of a head tilt–chin lift maneuver to open the airway in a trauma patient with suspected cervical spine injury. However, if providers are unable to open the airway and deliver effective ventilations using a jaw thrust, given the importance of a patent airway, using a head tilt–chin lift maneuver is recommended.
Figures 4, 5, 6, and 7 show, respectively, an infographic for pediatric BLS for lay rescuers, the current pediatric BLS algorithms for healthcare provider, single-rescuer CPR and 2-rescuer CPR, and the current algorithm for pediatric cardiac arrest.
Most pediatric cardiac arrests are triggered by respiratory deterioration. Airway management and effective ventilation are fundamental to pediatric resuscitation. Although the majority of patients can be successfully ventilated with bag-mask ventilation, this method requires interruptions in chest compressions and is associated with risk of aspiration and barotrauma.
Advanced airway interventions, such as supraglottic airway (SGA) placement or endotracheal intubation (ETI), may improve ventilation, reduce the risk of aspiration, and enable uninterrupted compression delivery. However, airway placement may interrupt the delivery of compressions or result in a malpositioned device. Advanced airway placement requires specialized equipment and skilled providers, and it may be difficult for professionals who do not routinely intubate children.
COR | LOE | Recommendation |
---|---|---|
2a | C-LD |
|
Recommendation-Specific Supportive Text
- A clinical trial and 2 propensity-matched retrospective studies show that ETI and bag-mask ventilation achieve similar rates of survival with good neurological function and survival to hospital discharge in pediatric patients with OHCA.1–3 Propensity-matched retrospective studies also show similar rates of survival with good neurological function and survival to discharge when comparing SGA with bag-mask ventilation in pediatric OHCA.2,3 No difference was observed in outcomes between SGA and ETI.2,3 There are limited data to compare outcomes between bagmask ventilation versus ETI in the management of IHCA,4 and there are no hospital-based studies of SGA. The data are not sufficient to support a recommendation for advanced airway use in IHCA. There may be specific circumstances or populations in which early advanced airway interventions are beneficial.
This recommendation was reviewed in the “2019 American Heart Association Focused Update on Pediatric Advanced Life Support: An Update to the American Heart Association Guidelines for Cardiopulmonary Resuscitation and Emergency Cardiovascular Care.”5
Vasoactive agents, such as epinephrine, are used during cardiac arrest to restore spontaneous circulation by optimizing coronary perfusion and maintaining cerebral perfusion, but the benefit and optimal timing of administration remain unclear.1,2 Antiarrhythmics reduce the risk of recurrent ventricular fibrillation (VF) and pulseless ventricular tachycardia (pVT) following defibrillation and may improve defibrillation success. Routine use of sodium bicarbonate and calcium is not supported by current data.3-7 However, there are specific circumstances when their administration is indicated, such as electrolyte imbalances and certain drug toxicities.
Medication dosing for children is based on weight, which is often difficult to obtain in an emergency setting. There are numerous approaches to estimating weight when an actual weight cannot be obtained.8
COR | LOE | Recommendations |
---|---|---|
2a | C-LD | |
2a | C-LD |
|
2a | C-LD | |
2b | C-LD | |
3: Harm | B-NR | |
3: Harm | B-NR |
Recommendation-Specific Supportive Text
- There are limited data in pediatrics comparing epinephrine administration to no epinephrine administration in any setting. In an OHCA study of 65 children, 12 patients did not receive epinephrine due to lack of a route of administration, and only 1 child had ROSC.2 An OHCA study of 9 children who had cardiac arrest during sport or exertion noted a survival rate of 67%, of whom 83% did not receive epinephrine. All survivors received early chest compressions (within 5 minutes) and early defibrillation (within 10 minutes), and the initial cardiac arrest rhythm was a shockable rhythm.9 Intravenous/intraosseous (IV/IO) administration of epinephrine is preferred over ETT administration when possible.10,11
- One retrospective observational study of children with IHCA who received epinephrine for an initial nonshockable rhythm demonstrated that, for every minute delay in administration of epinephrine, there was a significant decrease in ROSC, survival at 24 hours, survival to discharge, and survival with favorable neurological outcome.12 Patients who received epinephrine within 5 minutes of CPR compared to those who received epinephrine more than 5 minutes after CPR initiation were more likely to survive to discharge.12 Four observational studies of pediatric OHCA demonstrated that earlier epinephrine administration increased rates of ROSC,13,14 survival to ICU admission,14 survival to discharge,14,16 and 30-day survival.15
- One observational study demonstrated an increased survival rate at 1 year in the group that was administered epinephrine at an interval of less than 5 minutes.17 One observational study of pediatric IHCA demonstrated that an average epinephrine administration interval of 5 to 8 minutes and of 8 to 10 minutes was associated with increased odds of survival compared with an epinephrine interval of 1 to 5 minutes.18 Both studies17,18 calculated the average interval of epinephrine doses by averaging all doses over total arrest time, which does not account for potential differences in dosing intervals throughout resuscitations of varying duration. No studies of pediatric OHCA on frequency of epinephrine dosing were identified.
- Two studies examined drug therapy of VF/pVT in infants and children.19,20 In Valdes et al, administration of lidocaine, but not amiodarone, was associated with higher rates of ROSC and survival to hospital admission.19 Neither lidocaine nor amiodarone significantly affected the odds of survival to hospital discharge; neurological outcome was not assessed. A propensity-matched study of an IHCA registry demonstrated no difference in outcomes for patients receiving lidocaine compared with amiodarone.20
- A recent evidence review identified 8 observational studies of sodium bicarbonate administration during cardiac arrest.5–7,21–25 Bicarbonate administration was associated with worse survival outcomes for both IHCA and OHCA. There are special circumstances in which bicarbonate is used, such as the treatment of hyperkalemia and sodium channel blocker toxicity, including from tricyclic antidepressants.
- Two observational studies examining the administration of calcium during cardiac arrest demonstrated worse survival and ROSC with calcium administration.4,23 There are special circumstances in which calcium administration is used, such as hypocalcemia, calcium channel blocker overdose, hypermagnesemia, and hyperkalemia.3
Recommendation 4 was reviewed in “2018 American Heart Association Focused Update on Pediatric Advanced Life Support: An Update to the American Heart Association Guidelines for Cardiopulmonary Resuscitation and Emergency Cardiovascular Care.”26
COR | LOE | Recommendations |
---|---|---|
1 | C-EO |
|
2b | B-NR |
|
2b | C-LD |
Weight-Based Dosing of Resuscitation Medications
- There are many theoretical concerns about the use of actual body weight (especially in overweight or obese patients).27–29 However, there are no data about the safety and efficacy of adjusting medication dosing in obese patients. Such adjustments could result in inaccurate dosing of medications.30,31
- Several studies suggest that inclusion of body habitus or anthropometric measurements further refines and improves weight estimations using length-based measures.8 However, there is considerable variation in these methods, and the training required to use these measures may not be practical in every context.
- Cognitive aids can assist in the accurate approximation of body weight (described as being within 10% to 20% of measured total body weight). Several recent studies demonstrated high variability of weight estimates, with a tendency toward underestimation of total body weight yet closely approximating ideal body weight.29,32,33
The risk of VF/pVT steadily increases throughout childhood and adolescence but remains less frequent than in adults. Cardiac arrest due to an initial rhythm of VF/pVT has better rates of survival to hospital discharge with favorable neurological function than cardiac arrests due to an initial nonshockable rhythm. Shockable rhythms may be the initial rhythm of the cardiac arrest (primary VF/pVT) or may develop during the resuscitation (secondary VF/pVT). Defibrillation is the definitive treatment for VF/pVT. The shorter the duration of VF/pVT, the more likely that the shock will result in a perfusing rhythm. Both manual defibrillators and AEDs can be used to treat VF/pVT in children. Manual defibrillators are preferred when a shockable rhythm is identified by a healthcare provider because the energy dose can be titrated to the patient’s weight. AEDs have high specificity in recognizing pediatric shockable rhythms. Biphasic, instead of monophasic, defibrillators are recommended because less energy is required to achieve termination of VF/pVT, with fewer side effects. Many AEDs are equipped to attenuate (reduce) the energy dose to make them suitable for infants and children younger than 8 years of age.
COR | LOE | Recommendations |
---|---|---|
2a | C-LD |
|
2b | C-LD |
|
2b | C-LD |
|
Recommendation-Specific Supportive Text
- 2, and 3. A systematic review1 demonstrated no relationship between energy dose and any outcome. No randomized controlled trials were available, and most studies only evaluated the first shock. An IHCA case series of 71 shocks in 27 patients concluded that 2 J/kg terminated VF, but neither the subsequent rhythm nor the outcome of the resuscitation was reported.2 A small case series of prolonged OHCA observed that 2 to 4 J/kg shock terminated VF 14 times in 11 patients, resulting in asystole or pulseless electric activity, with no survivors to hospital discharge.3 In 1 observational study of IHCA,4 a higher initial energy dose of more than 3 to 5 J/kg was less effective than 1 to 3 J/kg in achieving ROSC. Three small, observational studies of pediatric IHCA3,5 and OHCA6 found no specific initial energy dose that was associated with successful defibrillation. One study suggested that 2 J/kg was an ineffective dose, especially for secondary VF.7
COR | LOE | Recommendations |
---|---|---|
1 | C-EO |
|
1 | C-EO | |
1 | C-EO |
Recommendation-Specific Supportive Text
- There are currently no pediatric data available regarding the optimal timing of CPR prior to defibrillation. Adult studies demonstrate no benefit of a prolonged period of CPR prior to initial defibrillation.8–12
- There are currently no pediatric data concerning the best sequence for coordination of shocks and CPR. Adult studies comparing a 1-shock protocol versus a 3-shock protocol for treatment of VF suggest significant survival benefit with the single-shock protocol.13,14
- Prolonged pauses in chest compressions decrease blood flow and oxygen delivery to vital organs, such as the brain and heart, and are associated with lower survival.13,15
COR | LOE | Recommendations |
---|---|---|
1 | C-EO |
|
2b | C-LD | |
2b | C-LD |
|
Recommendation-Specific Supportive Text
- Larger pad or paddle size decreases transthoracic impedance, which is a major determinant of current delivery.16–18
- One human and 1 porcine study demonstrated no significant difference in shock success or ROSC when comparing anterior-lateral with anterior-posterior position.7,19
- One study demonstrated no significant difference in median time to shock with paddles compared with self-adhesive pads.20
COR | LOE | Recommendations |
---|---|---|
1 | C-LD |
|
1 | C-EO | |
2b | C-EO |
Recommendation-Specific Supportive Text
- Shockable rhythms are infrequent in infants.21,22 Studies of rhythm identification algorithms have demonstrated high specificity for shockable rhythms in infants and children.23–25 Although there are no direct comparisons between pediatric attenuator and nonattenuator AED-delivered shocks, multiple case reports and case series document shock success with survival when a pediatric attenuator was used.26–32
- There are no specific studies comparing manual defibrillators with AEDs in infants or children. Manual defibrillators are preferred for in-hospital use because the energy dose can be titrated to the patient’s weight. In adults, use of an AED in hospitals did not improve survival,33 and the perishock pauses needed for rhythm analysis were prolonged.34
- AEDs without pediatric modifications deliver 120 to 360 Joules, exceeding the recommended dose for children weighing less than 25 kg. However, there are reports of safe and effective AED use in infants and young children when the dose exceeded 2 to 4 J/kg.26–28,30,35 Because defibrillation is the only effective therapy for VF, an AED without a dose attenuator may be lifesaving.
Initiating and maintaining high-quality CPR is associated with improved rates of ROSC, survival, and favorable neurological outcome, yet measured CPR quality is often suboptimal.1–3 Noninvasive and invasive monitoring techniques may be used to assess and guide the quality of CPR. Invasive arterial blood pressure monitoring during CPR provides insight to blood pressures generated with compressions and medications.4 End-tidal CO2 (ETCO2) reflects both the cardiac output produced and ventilation efficacy and may provide feedback on the quality of CPR.5 A sudden rise in ETCO2 may be an early sign of ROSC.6 CPR feedback devices (ie, coaching, audio, and audiovisual devices) may improve compression rate, depth, and recoil within a system of training and quality assurance for high-quality CPR. Point of care ultrasound, specifically echocardiography, during CPR has been considered for identification of reversible causes of arrest. Technologies that are under evaluation to assess resuscitation quality include noninvasive measures of cerebral oxygenation, such as using near infrared spectroscopy during CPR.
COR | LOE | Recommendations |
---|---|---|
2a | C-LD |
|
2b | C-LD | |
2b | C-EO | |
2b | C-EO |
|
Recommendation-Specific Supportive Text
- A prospective observational study of pediatric patients with invasive arterial blood pressure monitoring during the first 10 minutes of CPR demonstrated higher rates of favorable neurological outcome if the diastolic blood pressure was at least 25 mm Hg in infants and at least 30 mm Hg in children.4 Of note, the cut points for diastolic blood pressure tracings were analyzed using post hoc waveform analysis; therefore, prospective evaluation is needed.
- A single-center, retrospective study of in-hospital CPR in infants found that ETCO2 values between 17 and 18 mm Hg had a positive predictive value for ROSC of 0.885.7 A prospective, multicenter observational study of IHCA did not find an association between mean ETCO2 and outcomes.8
- A simulation trial of pediatric healthcare providers demonstrated a significant improvement in chest compression depth and rate compliance when they received visual feedback (compared to no feedback), although overall compression quality remained poor.9 One small observational study of 8 children with IHCA did not find an association between CPR with or without audiovisual feedback and survival to discharge, although feedback decreased excessive compression rates.10
- Several case series evaluated the use of bedside echocardiography to identify reversible causes of cardiac arrest, including pulmonary embolism.11,12 One prospective observational study of children (without cardiac arrest) admitted to an ICU reported good agreement of estimates of shortening fraction and inferior vena cava volume between emergency physicians using bedside limited echocardiography and cardiologists performing formal echocardiography.13
Extracorporeal cardiopulmonary resuscitation (ECPR) is defined as the rapid deployment of venoarterial extracorporeal membrane oxygenation (ECMO) for patients who do not achieve sustained ROSC. It is a resource-intense, complex, multidisciplinary therapy that traditionally has been limited to large pediatric medical centers with providers who have expertise in the management of children with cardiac disease. Judicious use of ECPR for specific patient populations and within dedicated and highly practiced environments has proved successful, especially for IHCA with reversible causes.1 ECPR use rates have increased, with single-center reports in both adults and children suggesting that application of this therapy across broader patient populations may improve survival after cardiac arrest.2–4
There are no studies of ECPR demonstrating improved outcomes following pediatric OHCA.
Recommendation-Specific Supportive Text
- One observational registry study of ECPR for pediatric IHCA after cardiac surgery demonstrated that ECPR was associated with higher rates of survival to hospital discharge than conventional CPR.5 A propensity-matched analysis of ECPR compared with conventional CPR using the same registry found that ECPR was associated with favorable neurological outcome in patients with IHCA of any etiology.6 There is insufficient evidence to suggest for or against the use of ECPR for pediatric patients experiencing OHCA or pediatric patients with noncardiac disease experiencing IHCA refractory to conventional CPR.
This recommendation was reviewed in the “2019 American Heart Association Focused Update on Pediatric Advanced Life Support: An Update to the American Heart Association Guidelines for Cardiopulmonary Resuscitation and Emergency Cardiovascular Care.”7
Successful resuscitation from cardiac arrest results in a post–cardiac arrest syndrome that can evolve in the days after ROSC. The components of post–cardiac arrest syndrome are (1) brain injury, (2) myocardial dysfunction, (3) systemic ischemia and reperfusion response, and (4) persistent precipitating pathophysiology.1,2 Post–cardiac arrest brain injury remains a leading cause of morbidity and mortality in adults and children because the brain has limited tolerance of ischemia, hyperemia, or edema. Pediatric post–cardiac arrest care focuses on anticipating, identifying, and treating this complex physiology to improve survival and neurological outcomes.
Targeted temperature management (TTM) refers to continuous maintenance of patient temperature within a narrowly prescribed range while continuously monitoring temperature. All forms of TTM avoid fever, and hypothermic TTM attempts to treat reperfusion syndrome by decreasing metabolic demand, reducing free radical production, and decreasing apoptosis.2
Identification and treatment of derangements—such as hypotension, fever, seizures, acute kidney injury, and abnormalities of oxygenation, ventilation, and electrolytes—are important because they may impact outcomes.
COR | LOE | Recommendations |
---|---|---|
1 | A | |
2a | B-R |
Recommendation-Specific Supportive Text
- and 2. Two pediatric randomized clinical trials of TTM (32°C–34°C for 48 hours followed by 3 days of TTM 36°C–37.5°C versus TTM 36°C–37.5°C for a total of 5 days) after IHCA or OHCA in children with coma following ROSC found no difference in 1-year survival with a favorable neurological outcome.3,4 Hyperthermia was actively prevented with TTM. Continuous core temperature monitoring was used for the 5 days of TTM in both trials.
Recommendations 1 and 2 were reviewed in the “2019 American Heart Association Focused Update on Pediatric Advanced Life Support: An Update to the American Heart Association Guidelines for Cardiopulmonary Resuscitation and Emergency Cardiovascular Care.”5
COR | LOE | Recommendations |
---|---|---|
1 | C-LD |
|
1 | C-EO |
|
Recommendation-Specific Supportive Text
- and 2. Two observational studies demonstrated that systolic hypotension (below 5th percentile for age and sex) at approximately 6 to 12 hours following cardiac arrest is associated with decreased survival to discharge.6,7 Another observational study found that patients who had longer periods of hypotension within the first 72 hours of ICU post–cardiac arrest care had decreased survival to discharge.8 In an observational study of patients with arterial monitoring during and immediately after cardiac arrest, diastolic hypertension (above 90th percentile) in the first 20 minutes after ROSC was associated with an increased likelihood of survival to discharge.9 Because blood pressure is often labile in the post–cardiac arrest period, continuous arterial pressure monitoring is recommended.
COR | LOE | Recommendations |
---|---|---|
2b | C-LD |
|
2b | C-LD | |
2b | C-LD |
Recommendation-Specific Supportive Text
- and 2. Because an arterial oxyhemoglobin saturation of 100% may correspond to a Pao2 between 80 and approximately 500 mm Hg, it is reasonable to target an oxyhemoglobin saturation between 94% and 99%. Three small observational studies of pediatric IHCA and OHCA did not show an association between hyperoxemia and outcome.10,11,13 In a larger observational study of pediatric IHCA and OHCA patients, the presence of normoxemia compared with hyperoxemia after ROSC was associated with improved survival to pediatric ICU discharge.12
- One observational study demonstrated that both hypercapnia and hypocapnia after ROSC were associated with increased mortality.11 One small observational study demonstrated no association between hypercapnia (Paco2 greater than 50 mm Hg) or hypocapnia (Paco2 less than 30 mm Hg) and outcome.10 Another observational study of pediatric IHCA, showed hypercapnia (Paco2 50 mm Hg or greater) was associated with decreased survival to hospital discharge.14 Because hypercapnia and hypocapnia impact cerebral blood flow, normocapnia should be the focus after ROSC while accounting for patients who have chronic hypercapnia.
COR | LOE | Recommendations |
---|---|---|
1 | C-LD |
|
1 | C-LD | |
2a | C-EO |
Recommendation-Specific Supportive Text
- Nonconvulsive seizures and nonconvulsive status epilepticus are common after pediatric cardiac arrest.15–18 The American Clinical Neurophysiology Society recommends continuous EEG monitoring for encephalopathic patients after pediatric cardiac arrest.15 Nonconvulsive seizures and nonconvulsive status epilepticus cannot be detected without EEG monitoring.15
- and 3. There is insufficient evidence to determine whether treatment of convulsive or nonconvulsive seizures improves neurological and/or functional outcomes after pediatric cardiac arrest. Both convulsive and nonconvulsive status epilepticus are associated with worse outcomes.17 The Neurocritical Care Society recommends treating status epilepticus with the goal of stopping convulsive and electrographic seizure activity.19
Figure 8 shows the checklist for post–cardiac arrest care.
Early and reliable prognostication of neurological outcome in pediatric survivors of cardiac arrest is essential to guide treatment, enable effective planning, and provide family support. Clinicians use patient and cardiac arrest characteristics, postarrest neurological examination, laboratory results, neurological imaging (eg, brain computed tomography and MRI), and EEG to guide prognostication. At this time, no single factor or validated decision rule has been identified to reliably predict either favorable or unfavorable outcome within 24 to 48 hours of ROSC. EEG, neuroimaging, and serum biomarkers when used alone predict outcome with only moderate accuracy, and more data are needed before applying these to individual patients.
COR | LOE | Recommendations |
---|---|---|
2a | B-NR |
|
2a | B-NR | |
2a | B-NR |
|
Recommendation-Specific Supportive Text
- Eight retrospective observational studies demonstrate that EEG background patterns are associated with neurological outcomes at discharge.1–8 The presence of sleep spindles,3,4,8 normal background,2 and reactivity7,8 is associated with favorable outcomes. Burst suppression and flat or attenuated EEG patterns are associated with unfavorable neurological outcome.1,2,5,8 However, these associations do not reach the high degrees of sensitivity and specificity needed to use EEG as a stand-alone modality for neuroprognostication.
- Several studies demonstrate the association of clinical history, patient characteristics, physical examination, imaging, and biomarker data with neurological outcome following cardiac arrest.1,7,9–19 To date, no single factor has demonstrated sufficient accuracy to prognosticate outcome. Elevated serum lactate, pH, or base deficit measured within the first 24 hours after cardiac arrest are associated with unfavorable outcome;9,11,12,16–18,20,21 however, specific cutoff values are unknown.
- Shorter submersion times are associated with better outcomes after pediatric nonfatal drowning.22–25 There is no clear association between patient age,23,26–31,38 water type,30,32,33 water temperature,23,25,34,35 emergency medical services response times35,36 or witnessed status,36–39 and neurological outcome following nonfatal drowning. No single factor accurately predicts prognosis after nonfatal drowning.
Survivors are at significant risk for both short-term and long-term physical, neurological, cognitive, emotional, and social morbidity.3 Many children who survive a cardiac arrest with a grossly “favorable outcome” have more subtle and sustained neuropsychological impairment.4 The full impact of brain injury on children’s development may not be fully appreciated until months to years after the cardiac arrest. Furthermore, because children are raised by caregivers, the impact of morbidity following cardiac arrest affects not only the child but also the family.
Recovery has been introduced as the sixth link in the Chain of Survival to acknowledge that survivors of cardiac arrest may require ongoing integrated medical, rehabilitative, caregiver, and community support in the months to years after their cardiac arrest (see Figure 9).3 Recent scientific statements from the AHA and ILCOR highlight the importance of studying long-term neurological and health-related quality-of-life outcomes.5,6
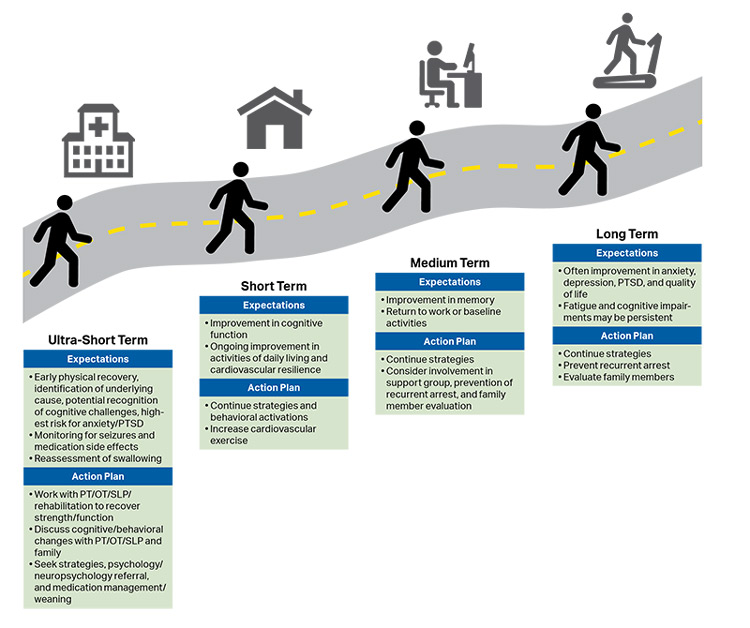
COR | LOE | Recommendations |
---|---|---|
1 | C-LD | |
2a | C-LD |
Recommendation-Specific Supportive Text
- Two randomized controlled trials of TTM for comatose children after IHCA or OHCA with a primary outcome of neurobehavioral outcome at 1 year7,8 showed that new morbidity is common.9–11 Many children who survived to 1 year with a favorable neurobehavioral outcome on Vineland Adaptive Behavior Scales-II (VABS-II) had global cognitive impairment or selective neuropsychological deficits.4
- Two randomized controlled trials of TTM for pediatric cardiac arrest demonstrated that neurological function improves for some survivors during the first year after cardiac arrest.10,11 Several case series of longer-term outcomes (more than 1 year after cardiac arrest) demonstrate ongoing cognitive, physical, and neuropsychological impairments.12–14 Recent statements from the AHA highlight the importance of follow-up after discharge, because patient recovery continues during the first year after cardiac arrest.3,5,6,15 It is unclear what impact ongoing childhood development has on recovery following pediatric cardiac arrest.
Over the past 20 years, the practice of maintaining family presence during resuscitation has increased. Most parents surveyed indicate that they would desire to be present during their child’s resuscitation. Older data suggest a lower incidence of anxiety and depression and more constructive grief behaviors among parents who were present when their child died.1
COR | LOE | Recommendations |
---|---|---|
1 | B-NR |
|
1 | B-NR | |
1 | C-LD |
Recommendation-Specific Supportive Text
- Qualitative studies generally show that there can be benefits for families if they are permitted to be present during the resuscitation of their children. Parents stated that they believed their presence brought their child comfort and that it helped them to adjust to the loss of their child.2 Other surveys of parents reported that they desired to be present to understand what was happening, to know that all that could be done was being done, and to keep physical contact with their child.3,4 However, not all parents who were present for their child’s resuscitation would choose to do so again.5 Some concerns have been raised about family presence during resuscitation, such as trauma for the family, interference with procedures, impact on technical performance, and concern for teaching and clinical decision-making, but these have not been supported by the available evidence.6–8 Experienced providers are more likely than trainees to support family presence.9,10
- The presence of a facilitator to support the family is helpful.11,12 It is important that the family have a dedicated team member during the resuscitation to help process the traumatic event, but this is not always feasible. Lack of an available facilitator should not prevent family presence at the resuscitation.
- Most surveys indicate family presence is not disruptive during resuscitation, although some providers feel increased stress.13 Providers with significant experience with family presence acknowledge occasional negative experiences.14
Hypertrophic cardiomyopathy, coronary artery anomalies, and arrhythmias are common causes of sudden unexplained cardiac arrest in infants and children. Up to one third of young patients who do not survive sudden unexplained cardiac arrest have no abnormalities found on gross and microscopic autopsy.1–4 Postmortem genetic evaluation (“molecular autopsy”) is increasingly used to inform etiology of sudden unexplained cardiac arrest.5 In addition to providing an explanation for the arrest, genetic diagnosis can identify inheritable cardiac disease, such as channelopathy and cardiomyopathy, enabling screening and preventive measures for relatives.
COR | LOE | Recommendations |
---|---|---|
1 | C-EO |
|
1 | C-EO | |
1 | C-EO |
|
Recommendation-Specific Supportive Text
- In 7 cohort studies, mutations causing channelopathies were identified in 2% to 10% of infants with sudden infant death syndrome.6–12 Among children and adolescents with sudden unexplained cardiac arrest and a normal autopsy, 9 cohort studies report identification of genetic mutations associated with channelopathy or cardiomyopathy.13–21
- In 7 cohort studies17,18,20,22–25 and 1 population-based study21 of screening using clinical and laboratory (electrocardiographic, molecular genetic screening) investigations, 14% to 53% of first- and second-degree relatives of patients with sudden unexplained cardiac arrest had inherited, arrhythmogenic disorders. In 7 cohort studies, mutations causing channelopathies were identified in 2% to 10% of infants with sudden infant death syndrome.6–12
- Several cohort studies report the utility of obtaining a complete past medical and family history after sudden unexplained cardiac arrest as well as review of prior electrocardiograms. A small case series suggested that specific genetic screening of family members was directed by the clinical history.20 Three small cohort studies and 1 population- based study reported relevant clinical symptoms or medical comorbidities, such as seizure, syncope, palpitations, chest pain, left arm pain, and shortness of breath, among patients who had a sudden unexplained cardiac arrest and their family members.16,17,19,21
Shock is the failure of oxygen delivery to meet tissue metabolic demands and can be life threatening. The most common type of pediatric shock is hypovolemic, including shock due to hemorrhage. Distributive, cardiogenic, and obstructive shock occur less frequently. Often, multiple types of shock can occur simultaneously; thus, providers should be vigilant. Cardiogenic shock in its early stages can be difficult to diagnose, so a high index of suspicion is warranted.
Shock progresses over a continuum of severity, from a compensated to a decompensated (hypotensive) state. Compensatory mechanisms include tachycardia and increased systemic vascular resistance (vasoconstriction) in an effort to maintain cardiac output and end-organ perfusion. As compensatory mechanisms fail, hypotension and signs of inadequate end-organ perfusion develop, such as depressed mental status, decreased urine output, lactic acidosis, and weak central pulses.
Early administration of intravenous fluids to treat septic shock has been widely accepted based on limited evidence. Mortality from pediatric sepsis has declined in recent years, concurrent with implementation of guidelines emphasizing the role of early antibiotic and fluid administration.1 Controversies in the management of septic shock include volume of fluid administration and how to assess the patient’s response, the timing and choice of vasopressor agents, the use of corticosteroids, and modifications to treatment algorithms for patients in sepsis-related cardiac arrest. Previous AHA guidelines2 have considered large studies of patients with malaria, sickle cell anemia, and dengue shock syndrome; however, these patients require special consideration that make generalization of results from these studies problematic.
Resuscitation guidance for children with hemorrhagic shock is evolving, as crystalloid-then-blood paradigms are being challenged by resuscitation protocols using blood products early in resuscitation. However, the ideal resuscitation strategy for a given type of injury is often unknown.
COR | LOE | Recommendations |
---|---|---|
1 | C-LD |
|
2a | B-R |
|
2a | B-NR |
|
2a | C-LD |
|
Recommendation-Specific Supportive Text
- Although fluids remain the mainstay initial therapy for infants and children in shock, especially in hypovolemic and septic shock, fluid overload can lead to increased morbidity.3 In 2 randomized trials of patients with septic shock, those who received higher fluid volumes4 or faster fluid resuscitation5 were more likely to develop clinically significant fluid overload characterized by increased rates of mechanical ventilation and worsening oxygenation.
- In a systematic review, 12 relevant studies were identified, though 11 assessed colloid or crystalloid fluid resuscitation in patients with malaria, dengue shock syndrome, or “febrile illness” in sub-Saharan Africa.6 There was no clear benefit to crystalloid or colloid solutions as first-line fluid therapy in any of the identified studies.
- One pragmatic, randomized controlled trial compared the use of balanced (lactated Ringer’s solution) to unbalanced (0.9% saline) crystalloid solutions as the initial resuscitation fluid and showed no difference in relevant clinical outcomes.7 A matched retrospective cohort study of pediatric patients with septic shock showed no difference in outcomes,8 though a propensity-matched database study showed an association with increased 72-hour mortality and vasoactive infusion days with unbalanced crystalloid fluid resuscitation.9
- In a small, randomized controlled study, there were no significant differences in outcomes with the use of 20 mL/kg as the initial fluid bolus volume (compared with 10 mL/kg); however, the study was limited by a small sample size.4
COR | LOE | Recommendations |
---|---|---|
2a | C-LD | |
2a | C-EO |
|
2b | B-NR | |
2b | C-LD |
|
Recommendation-Specific Supportive Text
- Two randomized controlled trials comparing escalating doses of dopamine or epinephrine demonstrated improvement in timing of resolution of shock10 and 28-day mortality11 with the use of epinephrine over dopamine. Both studies were conducted in resource-limited settings, and the doses of inotropes used may not have been directly comparable, limiting conclusions from the studies. Medications that increase systemic vascular resistance, such as norepinephrine, may also be a reasonable initial vasopressor therapy in septic shock patients.1,12–14 Recent international sepsis guidelines recommend the choice of the medications to be guided by patient physiology and clinician preferences.1
- No studies support deviations from standard life-support algorithms to improve outcomes in patients with sepsis-associated cardiac arrest. Sepsis-associated cardiac arrest is associated with worse outcomes than other causes of cardiac arrest.15
- A meta-analysis20 showed no change in survival with corticosteroid use in pediatric septic shock, though a more recent randomized controlled trial suggested a shorter time to reversal of shock with steroid use.17 Two observational studies18,19 suggested there may be specific subpopulations, based on genomics, that would either benefit or experience harm from steroid administration, though these subpopulations are difficult to identify clinically. Patients at risk for adrenal insufficiency (eg, those on chronic steroids, patients with purpura fulminans) are more likely to benefit from steroid therapy.12
- In situations when epinephrine or norepinephrine are not available, dopamine is a reasonable alternative initial vasoactive infusion in patients with fluid-refractory septic shock.10,11 Patients with vasodilatory shock may require a higher dose of dopamine.12
COR | LOE | Recommendations |
---|---|---|
1 | C-EO |
|
2b | C-EO |
|
Recommendation-Specific Supportive Text
- and 2. Cardiogenic shock in infants and children is uncommon and associated with high mortality rates. No studies were identified comparing outcomes between vasoactive medications. For patients with hypotension, medications such as epinephrine may be more appropriate as an initial inotropic therapy. Because of the rarity and complexity of these presentations, expert consultation is recommended when managing infants and children in cardiogenic shock.
COR | LOE | Recommendation |
---|---|---|
2a | C-EO |
|
Recommendation-Specific Supportive Text
- There are no prospective pediatric data comparing the administration of early blood products versus early crystalloid for traumatic hemorrhagic shock. A scoping review identified 6 recent retrospective studies that compared patient outcomes with the total volume of crystalloid resuscitation received in the first 24 to 48 hours among children with hemorrhagic shock.21–25,28 Four studies reported no differences in survival to 24 hours, survival at 30 days with good neurological outcome, or survival to discharge.21,24,25,28 Large-volume resuscitation was associated with increased hospital/ICU length of stay in 5 of the 6 studies.22–25,28 One study reported lower survival to hospital discharge among children who received more than 60 mL/kg crystalloid compared to lower volume groups.22 Despite limited pediatric data, recent guidelines for adults from the Eastern Association for the Surgery of Trauma,26 the American College of Surgeons, and the National Institute for Health and Care Excellence27 suggest the early use of balanced ratios of packed red blood cells, fresh frozen plasma, and platelets for trauma-related hemorrhagic shock.29
Respiratory failure occurs when a patient’s breathing becomes inadequate and results in ineffective oxygenation and ventilation. This can occur due to disordered control of breathing, upper airway obstruction, lower airway obstruction, respiratory muscle failure, or parenchymal lung disease. Providing assisted ventilation when breathing is absent or inadequate, relieving foreign body airway obstruction (FBAO), and administering naloxone in opioid overdose can be lifesaving.
Suffocation (eg, FBAO) and poisoning are leading causes of death in infants and children. Balloons, foods (eg, hot dogs, nuts, grapes), and small household objects are the most common causes of FBAO in children,1–3 whereas liquids are common among infants.4 It is important to differentiate between mild FBAO (the patient is coughing and making sounds) and severe FBAO (the patient cannot make sounds). Patients with mild FBAO can attempt to clear the obstruction by coughing, but intervention is required in severe obstruction.
In the United States in 2017, opioid overdose caused 79 deaths in children less than 15 years old and 4094 deaths in people age 15 to 24 years.5 Naloxone reverses the respiratory depression of narcotic overdose,6 and, in 2014, the US Food and Drug Administration approved the use of a naloxone autoinjector by lay rescuers and healthcare providers. Naloxone intranasal delivery devices are also available.
COR | LOE | Recommendations |
---|---|---|
1 | C-EO |
|
2a | C-EO |
|
Recommendation-Specific Supportive Text
- and 2. There are no pediatric-specific clinical studies evaluating the effect of different ventilation rates on outcomes in inadequate breathing with a pulse. One multicenter observational study found that high ventilation rates (at least 30/min in children younger than 1 year of age, at least 25/min in children older than 1 year) during CPR with an advanced airway for cardiac arrest were associated with improved ROSC and survival.7 For the ease of training, the suggested respiratory rate for the patient with inadequate breathing and a pulse has been increased from 1 breath every 3 to 5 seconds to 1 breath every 2 to 3 seconds to be consistent with the new CPR guideline recommendation for ventilation in patients with an advanced airway.
COR | LOE | Recommendations |
---|---|---|
1 | C-LD | |
1 | C-LD | |
1 | C-LD | |
1 | C-LD |
|
1 | C-LD |
|
3: Harm | C-LD |
|
Recommendation-Specific Supportive Text
- and 2. There are no high-quality data to support recommendations regarding FBAO in children. Many FBAOs are relieved by allowing the patient to cough or, if severe, are treated by bystanders using abdominal thrusts.4,8,9
- Observational data primarily from case series support the use of back blows4,9,10 or chest compressions10,11 for infants. Abdominal thrusts are not recommended for infants given the potential to cause abdominal organ injury.12
- Once the victim is unconscious, observational data support immediate provision of chest compressions whether or not the patient has a pulse.11
- and 6. Observational data suggest that the risk of blind finger sweeps outweighs any potential benefit in the management of FBAO.13–15
COR | LOE | Recommendations |
---|---|---|
1 | C-LD | |
1 | C-EO | |
1 | C-EO | |
2a | B-NR |
|
Recommendation-Specific Supportive Text
- Initial management should focus on support of the patient’s airway and breathing. This begins with opening the airway followed by delivery of rescue breaths, ideally with the use of a bag-mask or barrier device.17,18 Provision of life support should continue if return of spontaneous breathing does not occur.
- Because there are no studies demonstrating improvement in patient outcomes from administration of naloxone during cardiac arrest, provision of CPR should be the focus of initial care.20 Naloxone can be administered along with standard advanced cardiovascular life support care if it does not delay components of high-quality CPR.
- Early activation of the emergency response system is critical for patients with suspected opioid overdose. Rescuers cannot be certain that the person’s clinical condition is due to opioid-induced respiratory depression alone. This is particularly true in first aid and BLS, where determination of the presence of a pulse is unreliable.21,22 Naloxone is ineffective in other medical conditions, including overdose involving nonopioids and cardiac arrest from any cause. Patients who respond to naloxone administration may develop recurrent central nervous system and/or respiratory depression and require longer periods of observation before safe discharge.37–40
- Twelve studies examined the use of naloxone in respiratory arrest, of which 5 compared intramuscular, intravenous, and/or intranasal routes of naloxone administration (2 RCT23,24 and 3 non-RCT25–27) and 9 assessed the safety of naloxone use or were observational studies of naloxone use.28–36 These studies report that naloxone is safe and effective in treatment of opioid-induced respiratory depression and that complications are rare and dose related.
These recommendations were taken from Part 3: Adult Basic and Advanced Life Support41 and further supported by a 2020 ILCOR evidence update.42 There were no pediatric data supporting these recommendations; however, due to the urgency of the opioid crisis, the adult recommendations should be applied to children.
Figures 10 and 11 are algorithms for opioid-associated emergencies for lay responders and healthcare providers.
It is important to select appropriate equipment and medications for pediatric intubation. Uncuffed ETTs were historically preferred for young children because the normal pediatric airway narrows below the vocal cords, creating an anatomic seal around the distal tube. In the acute setting and with poor pulmonary compliance, uncuffed ETTs may need to be changed to cuffed ETTs. Cuffed tubes improve capnography accuracy, reduce the need for ETT changes (resulting in high-risk reintubations or delayed compressions), and improve pressure and tidal volume delivery. However, high pressure in the cuff can cause airway mucosal damage. Although several studies have identified that cuffed tube use may actually decrease airway trauma by decreasing tube changes, attention must be made to selecting the correct tube size and cuff inflation pressure.1 ETT cuff pressures are dynamic during transport at altitude2 and with increasing airway edema.
Intubation is a high-risk procedure. Depending on the patient’s hemodynamics, respiratory mechanics, and airway status, the patient can be at increased risk for cardiac arrest during intubation. Therefore, it is important to provide adequate resuscitation before intubation.
Cricoid pressure during bag-mask ventilation and intubation has historically been used to minimize the risk of gastric contents refluxing into the airway, but there are concerns that tracheal compression may impede effective bag-mask ventilation and intubation success.
Confirmation of ETT placement in patients with a perfusing rhythm is not reliably achieved by auscultation of breath sounds, mist in the tube, or chest rise. Either colorimetric detector or capnography (ETCO2) can be used to assess initial ETT placement. In patients with decreased pulmonary blood flow from low cardiac output or cardiac arrest, ETCO2 may not be as reliable.
COR | LOE | Recommendations |
---|---|---|
1 | C-EO |
|
2a | C-LD |
|
Recommendation-Specific Supportive Text
- A retrospective study including 2953 children noted that, with 25 cm H2O of pressure to the airway and a slight leak around the ETT, there were no cases of clinically significant subglottic stenosis, and the incidence of stridor requiring reintubation was less than 1%.3
- Three systematic reviews, 2 randomized controlled trials, and 2 retrospective reviews support the safety of cuffed ETTs and the decreased need for ETT changes.4–10 These studies were almost entirely performed in the perioperative patient population, and intubation was performed by highly skilled airway providers. Thus, ETT duration may have been shorter than in critically ill patients. The use of cuffed ETTs is associated with lower reintubation rates, more successful ventilation, and improved accuracy of capnography without increased risk of complications.7,9–13 Cuffed ETTs may decrease the risk of aspiration.14,15
COR | LOE | Recommendations |
---|---|---|
2b | C-LD | |
3: No Benefit | C-LD | |
3: Harm | C-LD |
Recommendation-Specific Supportive Text
- 2, and 3. A retrospective, propensity score–matched study from a large pediatric ICU intubation registry showed that cricoid pressure during induction and bag-mask ventilation before tracheal intubation was not associated with lower rates of regurgitation.17 A study from the same pediatric ICU database reported external laryngeal manipulation was associated with lower initial tracheal intubation success.16
COR | LOE | Recommendations |
---|---|---|
2b | C-LD | |
2b | C-LD |
|
Recommendation-Specific Supportive Text
- The 2019 French Society of Anesthesia and Intensive Care Medicine guidelines state that atropine “should probably” be used as a preintubation drug in children 28 days to 8 years with septic shock, with hypovolemia, or with succinylcholine administration.18,19
- One nonrandomized, single-center intervention study did not identify an association between atropine dosing less than 0.1 mg and bradycardia or arrhythmias.20
COR | LOE | Recommendations |
---|---|---|
1 | C-LD |
|
2a | C-LD |
Recommendation-Specific Supportive Text
- Although there are no randomized controlled trials linking use of ETCO2 detection with clinical outcomes, the Fourth National Audit Project of the Royal College of Anesthetists and Difficult Airway Society concluded that the failure to use or inability to properly interpret capnography contributed to adverse events, including ICU-related deaths (mixed adult and pediatric data).21,22 One small randomized study showed that capnography was faster than clinical assessment in premature newborns intubated in the delivery room.23 There was no difference in patient outcomes between qualitative (colorimetric) and quantitative (capnography or numeric display) ETCO2 detectors.24–27
- Adult literature suggests monitoring and correct interpretation of capnography in intubated patients may prevent adverse events.21,22,28 This has been demonstrated in simulated pediatric scenarios, in which capnography increased provider recognition of possible ETT dislodgement.29,30
Bradycardia associated with hemodynamic compromise, even with a palpable pulse, may be a harbinger for cardiac arrest. As such, bradycardia with a heart rate of less than 60 beats per minute requires emergent evaluation for cardiopulmonary compromise. If cardiopulmonary compromise is present, the initial management in the pediatric patient requires simultaneous assessment of the etiology and treatment by supporting airway, ventilation, and oxygenation. If bradycardia with cardiopulmonary compromise is present despite effective oxygenation and ventilation, CPR should be initiated immediately. Outcomes are better for children who receive CPR for bradycardia before progressing to pulseless arrest.1 Correctable factors that contribute to bradycardia (ie, hypoxia, hypotension, hypoglycemia, hypothermia, acidosis, or toxic ingestions) should be identified and treated immediately.
COR | LOE | Recommendations |
---|---|---|
1 | C-LD | |
1 | C-LD | |
1 | C-EO | |
2b | C-LD |
|
Recommendation-Specific Supportive Text
- Two adult studies2,4 and 2 pediatric studies6,7 demonstrate that atropine is effective to treat bradycardia due to vagal stimulation, atrioventricular block, and intoxication. There is no evidence that atropine should be used for bradycardia due to other causes.
- Two retrospective analyses from the same database showed children who received CPR for bradycardia and poor perfusion had better outcomes than children who suffered pulseless cardiac arrest and received CPR.1,10 The longer the time between the initiation of CPR for bradycardia and the loss of a pulse, the lower the chance of survival.
- There are limited pediatric data regarding the treatment of bradycardia. A recent retrospective, propensity-matched study of pediatric patients with bradycardia with a pulse found that patients who received epinephrine had worse outcomes than patients who did not receive epinephrine.11 However, due to limitations of the study, further research on the impact of epinephrine on patients with bradycardia and a pulse is required.
- There are limited data about transcutaneous pacing for refractory bradycardia in children.12–16 In patients with complete heart block or sinus node dysfunction, especially when caused by congenital or acquired heart disease, emergency transcutaneous pacing may be considered. Pacing is not useful for asystole or bradycardia due to postarrest hypoxic or ischemic myocardial insult or respiratory failure.
Figure 12 shows the algorithm for pediatric bradycardia with a pulse.
Regular, narrow-complex tachyarrhythmias (QRS duration 0.09 seconds or less) are most commonly caused by re-entrant circuits, although other mechanisms (eg, ectopic atrial tachycardia, atrial fibrillation) sometimes occur. Regular, wide-complex tachyarrhythmias (greater than 0.09 seconds) can have multiple mechanisms, including supraventricular tachycardia (SVT) with aberrant conduction or ventricular tachycardia.
The hemodynamic impact of SVT in the pediatric patient can be variable, with cardiovascular compromise (ie, altered mental status, signs of shock, hypotension) occurring in the minority of patients. In hemodynamically stable patients, re-entrant SVT can often be terminated with vagal maneuvers.1,2 Adenosine remains the preferred medication to treat SVT in infants and children with a palpable pulse who do not respond to vagal maneuvers. For patients with hemodynamically stable wide-complex tachycardia and those in whom SVT recurs after initial successful treatment, expert consultation is important to diagnose etiology and customize treatment.
In hemodynamically unstable patients with SVT or wide-complex tachycardia, synchronized cardioversion should be considered.
COR | LOE | Recommendations |
---|---|---|
1 | C-LD |
|
1 | C-EO | |
2a | C-LD | |
2a | C-LD |
|
2b | C-LD |
Recommendation-Specific Supportive Text
- Intravenous adenosine remains generally effective for terminating re-entrant SVT within the first 2 doses.3–6 Of 5 retrospective observational studies on the management of tachyarrhythmias (4 single center, 1 multicenter), none directly compared adenosine to other drugs.6–9,17
- For patients with hemodynamically stable SVT that is refractory to vagal maneuvers or adenosine, consideration of alternative second-line agents should be guided by expert consultation, given potential proarrhythmic and life-threatening hemodynamic collapse with the administration of multiple antiarrhythmic agents. Multiple medications have been used as second-line agents for the management of adenosine-refractory SVT, including intravenous verapamil, β-blockers, amiodarone, procainamide, and sotalol.5–15,17 Few comparative studies exist.
- Vagal maneuvers are noninvasive, have few adverse effects, and effectively terminate SVT in many cases; exact success rates for each type of maneuver (ie, ice water to face, postural modification) are unknown.4 Although improved success rates have been reported with a postural modification to the standard Valsalva maneuver in adults,1 published pediatric experience with this technique is very limited. Upside-down positioning may be an additional form of a vagal maneuver that is effective in children.2
- Direct current synchronized cardioversion remains the treatment of choice for patients with hemodynamically unstable SVT (ie, with cardiovascular compromise characterized by altered mental status, signs of shock, or hypotension) and those with SVT unresponsive to standard measures. However, these cases are uncommon, and there are few data reporting outcomes from cardioversion of SVT.5,8,15 Consider administering sedation prior to synchronized cardioversion if resources are available and definitive therapy is not delayed.
- Procainamide and amiodarone are moderately effective treatments for adenosine-resistant SVT.12 There may be a small efficacy advantage favoring procainamide; adverse effects are frequent with both therapies. Intravenous sotalol was approved by the US Food and Drug Administration for the treatment of SVT in 2009. Only 3 reports describe its use in acute or subacute supraventricular tachyarrhythmias, with a 60% to 100% termination rate of SVT and atrial tachyarrhythmias.9,13,14 In the aforementioned studies, IV sotalol was administered under the guidance of pediatric electrophysiologists in the critical care or pediatric cardiology unit. Due to its potential proarrhythmic properties, it is unknown whether IV sotalol can be safely given in other settings. There is currently insufficient evidence in support for or against the use of IV sotalol for refractory SVT.
COR | LOE | Recommendations |
---|---|---|
1 | C-LD |
|
2a | C-EO |
|
Recommendation-Specific Supportive Text
- The occurrence of wide-complex tachycardia (QRS duration more than 0.09 s) with a pulse is rare in children and may originate from either the ventricle (ventricular tachycardia) or atria (SVT with aberrant conduction).18 Both pediatric and adult studies have identified potential populations at risk of proarrhythmic complications from antiarrhythmic therapies, including patients with underlying cardiomyopathies, long-QT syndrome, Brugada syndrome, and Wolff-Parkinson-White syndrome.19–23
- Electric direct current synchronized cardioversion should be provided urgently for the treatment of children with wide-complex tachycardia of either atrial or ventricular origin who are hemodynamically unstable with a pulse. Cardiovascular compromise is a key factor in determining the use of electric therapy instead of primary pharmacological management. There is insufficient evidence describing the incidence of wide-complex tachycardias with a pulse and hemodynamic stability, and there is no support for or against the use of specific antiarrhythmic drugs in the management of children with wide-complex tachycardia with a pulse.
Figure 13 shows the algorithm for pediatric tachycardia with a pulse.
Fulminant myocarditis can result in decreased cardiac output with end-organ compromise; conduction system disease, including complete heart block; and persistent supraventricular or ventricular arrhythmias, which can ultimately result in cardiac arrest.1 Because patients can present with nonspecific symptoms such as abdominal pain, diarrhea, vomiting, or fatigue, myocarditis can be confused with other, more common disease presentations. Outcomes can be optimized by early diagnosis and prompt intervention, including ICU monitoring and therapy. Sudden onset of heart block and multifocal ventricular ectopy in the patient with fulminant myocarditis should be considered a prearrest state. Treatment with external or intracardiac pacing or antiarrhythmic drugs may not be successful, and early transfer to a center capable of providing extracorporeal life support (ECLS) or mechanical circulatory support (MCS), such as temporary or implanted ventricular assist devices, is recommended.2,3
Noninfectious causes of cardiomyopathy in children include dilated cardiomyopathy, hypertrophic cardiomyopathy, restrictive cardiomyopathy, and miscellaneous (rare) forms of cardiomyopathy that include arrhythmogenic right ventricular dysplasia and mitochondrial and left ventricular noncompaction cardiomyopathies. Cardiomyopathy patients who present in acute decompensated heart failure refractory to mechanical ventilation and vasoactive administration have undergone preemptive MCS in the form of ECMO, short-term percutaneous ventricular assist device, or long-term implantable ventricular assist device prior to or during cardiac arrest.4,5
For patients who have worsening clinical status or incessant ventricular arrhythmias, ECLS can be lifesaving when initiated prior to cardiac arrest. ECLS also offers an opportunity to wean inotropic support, assist myocardial recovery, and serve as a bridge to cardiac transplantation if needed. The use of ECLS and MCS have improved outcomes from acute myocarditis, with a high possibility of partial or complete recovery of myocardial function.2,6
COR | LOE | Recommendations |
---|---|---|
1 | C-LD | |
2a | B-NR | |
2a | B-NR |
|
Recommendation-Specific Supportive Text
- Three retrospective studies have evaluated predictors of worse outcome in fulminant myocarditis, noting increased incidence of cardiac arrest and the need for ECLS in this high-risk population.1,7,8 In 1 study, nearly half of fulminant myocarditis patients required CPR, and nearly one third received MCS.7 Even modest decreases in left ventricular ejection fraction are associated with the need for invasive circulatory support.8
- The prognosis for patients with fulminant myocarditis who receive ECLS or MCS can be good. In 1 study, 13 (46%) of 28 children requiring MCS survived without transplant.9 One study noted that outcomes for ECPR patients cannulated with a diagnosis of myocarditis are superior to other arrest and illness categories leading to ECPR (ie, patients without congenital heart disease), noting myocarditis as a precannulation factor associated with improved survival.10 In the pre–cardiac arrest cardiomyopathy patient, newer forms of temporary circulatory support devices provide alternate and potentially improved support for decompensated heart failure requiring bridge to transplantation. These devices may provide a survival benefit over ECMO.4,5
- In 1 study, 95% of children with myocarditis who were placed on ECLS (n=15) or MCS (n=1) after cardiac arrest were alive 6 months later.9
The complexity and variability in pediatric congenital heart disease pose unique challenges during resuscitation. Children with single-ventricle heart disease typically undergo a series of staged palliative operations. The objectives of the first palliative procedure, typically performed during the neonatal period, are (1) to create unobstructed systemic blood flow, (2) to create an effective atrial communication to allow for atrial level mixing, and (3) to regulate pulmonary blood flow to prevent overcirculation and decrease the volume load on the systemic ventricle (Figure 14). During the second stage of palliation, a superior cavopulmonary anastomosis, or bidirectional Glenn/hemi-Fontan operation, is performed to create an anastomosis, which aids in the redistribution of systemic venous return directly to the pulmonary circulation (Figure 15). The Fontan is the final palliation, in which inferior vena caval blood flow is baffled directly to the pulmonary circulation, thereby making the single (systemic) ventricle preload dependent on passive flow across the pulmonary vascular bed (Figure 16).
Neonates and infants with single-ventricle physiology have an increased risk of cardiac arrest as a result of (1) increased myocardial work as a consequence of volume overload, (2) imbalances in relative systemic (Qs) and pulmonary (Qp) blood flow, and (3) potential shunt occlusion.1,2 Depending on the stage of repair, resuscitation may require control of pulmonary vascular resistance, oxygenation, systemic vascular resistance, or ECLS.
COR | LOE | Recommendations |
---|---|---|
2a | B-NR |
|
2a | C-LD |
|
2a | C-LD |
|
2a | C-LD | |
2a | C-EO |
|
Recommendation-Specific Supportive Text
- In the early postoperative period, noninvasively measured regional cerebral and somatic saturations, via near infrared spectroscopy, can predict outcomes of early mortality and ECLS use following stage I Norwood palliation. There are retrospective data that postoperative near infrared spectroscopy measures may be targets for goal-directed interventions.3
- Afterload reduction using vasodilators (sodium nitroprusside or phentolamine), with or without a phosphodiesterase type III inhibitor (eg, milrinone), reduces systemic vascular resistance, serum lactate, arterial venous oxygen difference, and the need for ECPR in the postoperative period for shunt-dependent single-ventricle patients.4,5
- In the period before single-ventricle palliation, cautious use of controlled hypoventilation can reduce Qp:Qs by increasing pulmonary vascular resistance, narrowing the arterial-venous oxygen difference, and increasing cerebral oxygen delivery. Simple hypoventilation can also increase the pulmonary vascular resistance but can be associated with unwanted atelectasis or respiratory acidosis.6,7
- For cardiac arrest before or after Stage I palliation repair, the use of ECPR is associated with improved survival. In 2 observational studies, 32% to 54% of neonates requiring ECPR survived, and, in 1 study, the odds of survival improved in cardiac arrest patients managed with ECPR.8,9
- Treatment of acute shunt obstruction can include administration of oxygen, vasoactive agents (eg, phenylephrine, norepinephrine, epinephrine) to maximize shunt perfusion pressure, anticoagulation with heparin (50–100 U/kg bolus), shunt intervention by catheterization or surgery, and ECLS.2
COR | LOE | Recommendations |
---|---|---|
2a | B-NR |
|
2b | B-NR |
|
Recommendation-Specific Supportive Text
- In patients immediately following bidirectional Glenn placement, a ventilation strategy with higher Paco2 improved oxygenation.10
- In 1 retrospective analysis of the Extracorporeal Life Support Organization database, among infants in whom a bidirectional Glenn had been placed and in whom ECLS was required, survival was similar in patients who had cardiac arrest before ECLS (16/39, 41%) and those who did not (26/64, 41%).11
These topics were reviewed previously in “Cardiopulmonary Resuscitation in Infants and Children With Cardiac Disease: A Scientific Statement From the American Heart Association.”12
Pulmonary hypertension is a rare disease in infants and children that is associated with significant morbidity and mortality. In the majority of pediatric patients, pulmonary hypertension is idiopathic or associated with chronic lung disease; congenital heart disease; and, rarely, other conditions, such as connective tissue or thromboembolic disease.1 Pulmonary hypertension occurs in 2% to 20% of patients following congenital heart disease surgery, with substantial morbidity and mortality.2 Pulmonary hypertension occurs in 2% to 5% of pediatric patients after cardiac surgery,3 and 0.7% to 5% of all cardiovascular surgical patients experience postoperative pulmonary hypertensive crises.4 Pulmonary hypertensive crises are acute rapid increases in pulmonary artery pressure accompanied by right-sided (or single-ventricle) heart failure. During pulmonary hypertensive crises, the right ventricle fails, and the increased afterload on the right ventricle produces increased myocardial oxygen demand at the same time that the coronary perfusion pressure and coronary blood flow decrease. The elevated left ventricle and right ventricle pressures lead to a fall in pulmonary blood flow and left-sided heart filling, with a resultant fall in cardiac output. Inotropic agents can be administered to improve right ventricle function, and vasopressors can be administered to treat systemic hypotension and improve coronary artery perfusion pressure. Once cardiac arrest has occurred, outcomes can be improved in the presence of an anatomic right-to-left shunt that permits left ventricle preload to be maintained without pulmonary blood flow.2 These crises are life threatening and may lead to systemic hypotension, myocardial ischemia, cardiac arrest, and death. Because acidosis and hypoxemia are both potent pulmonary vasoconstrictors, careful monitoring and management of these conditions are critical in the management of pulmonary hypertension. Treatment should also include the provision of adequate analgesics, sedatives, and muscle relaxants. Pulmonary vasodilators, including inhaled nitric oxide, inhaled prostacyclin, inhaled and intravenous prostacyclin analogs, and intravenous and oral phosphodiesterase type V inhibitors (eg, sildenafil) are used to prevent and treat pulmonary hypertensive crises.5–8
COR | LOE | Recommendations |
---|---|---|
1 | B-R | |
1 | B-NR |
|
1 | C-EO | |
2a | C-LD |
|
2b | C-LD |
Recommendation-Specific Supportive Text
- Treatment with inhaled nitric oxide reduces the frequency of pulmonary hypertensive crises and shortens time to extubation.9 In patients with atrioventricular septal defect repair and severe postoperative pulmonary hypertension, inhaled nitric oxide administration is associated with reduced mortality.7,10 Inhaled prostacyclin transiently produces pulmonary vasodilation and improves oxygenation, but the alkalinity of the drug can irritate airways, and precise dosing can be complicated by drug loss in the nebulization circuit.11,12
- Two physiological reviews and 1 randomized clinical trial have demonstrated that hypercarbia, hypoxemia, acidosis, atelectasis, and ventilation-perfusion mismatch can all lead to increases in pulmonary vascular resistance and, hence, elevation of pulmonary artery pressures in the immediate postoperative period.13–15
- Two observational studies looking at select high-risk postoperative cardiac patients found an attenuation in the stress response in those patients receiving fentanyl in the postoperative period.2,11,16,17
- Two physiological reviews and 1 randomized clinical trial have demonstrated that hypercarbia, hypoxemia, acidosis, atelectasis, and ventilation-perfusion mismatch can all lead to increases in pulmonary vascular resistance and, hence, elevation of pulmonary artery pressures in the immediate postoperative period.13–15
- ECLS has been used in children with pulmonary vascular disease after cardiopulmonary collapse or low cardiac output.18,19 Although outcomes remain poor in certain populations,20 advances in technology of extracorporeal devices may allow for bridging to MCS or to transplantation.21 Although patients with pulmonary hypertension who require ECLS have a high mortality rate, provision of ECLS can be lifesaving.11,22,23
These topics were reviewed previously in “Cardiopulmonary Resuscitation in Infants and Children With Cardiac Disease: A Scientific Statement From the American Heart Association”2 and “Pediatric Pulmonary Hypertension: Guidelines From the American Heart Association and American Thoracic Society.”11
Unintentional injuries are the most common cause of death among children and adolescents.1 Although many organizations have established trauma care guidelines,2–4 the management of traumatic cardiac arrest is often inconsistent. Cardiac arrest due to major blunt or penetrating injury in children has a very high mortality rate.5–8 Thoracic injury should be suspected in all thoracoabdominal trauma because tension pneumothorax, hemothorax, pulmonary contusion, or pericardial tamponade may impair hemodynamics, oxygenation, and ventilation.
COR | LOE | Recommendations |
---|---|---|
1 | C-EO | |
2b | C-LD |
|
Recommendation-Specific Supportive Text
- Early correction of reversible causes by reducing delays in the delivery of trauma-specific interventions may increase survival following penetrating traumatic cardiac arrest.9,10 Guidelines for cardiac arrest due to trauma recommend hemorrhage control, restoration of circulating blood volume, opening the airway, and relieving tension pneumothorax. These measures should be performed simultaneously with conventional resuscitation.
- Recent systematic reviews,11–14 multicenter retrospective studies,15,16 and single-center retrospective studies17 recommend emergent thoracotomy for pediatric patients who present pulseless after penetrating thoracic injury. There is no evidence to support emergent thoracotomy for infants and children with blunt injury who are without signs of life.12,18
During the literature review process, we identified several critical knowledge gaps related to pediatric basic and advanced life support. These topics are either current areas of ongoing research or lack significant pediatric evidence to support evidence-based recommendations. In addition, we identified topics for which systematic or scoping reviews are in process by the ILCOR Basic Life Support or Pediatric Life Support Task Forces and elected not to make premature recommendations until these reviews are available.
As is so often the case in pediatric medicine, many recommendations are extrapolated from adult data. This is particularly true for the BLS components of pediatric resuscitation. The causes of pediatric cardiac arrest are very different from cardiac arrest in adults, and pediatric studies are critically needed. Furthermore, infants, children, and adolescents are distinct patient populations. Dedicated pediatric resuscitation research is a priority given the more than 20 000 infants, children, and adolescents who suffer cardiac arrest in the United States each year.
Critical knowledge gaps are summarized in Table 2.
Table 2. Critical Knowledge Gaps Due to Insufficient Pediatric Data |
---|
What is the optimal method of medication delivery during CPR: IO or IV? |
What is the optimal method to determine body weight for medication administration? |
In what time frame should the first dose of epinephrine be administered during pulseless cardiac arrest? |
With what frequency should subsequent doses of epinephrine be administered? |
With what frequency should epinephrine be administered in infants and children during CPR who are awaiting ECMO cannulation? |
Are alternative compression techniques (cough CPR, fist pacing, interposed abdominal compression CPR) more effective alternatives to CPR? |
With what frequency should the rhythm be checked during CPR? |
What is the optimal method of airway management during OHCA–bag-mask ventilation, supraglottic airway, or endotracheal tube? |
What is the optimal FIO2 to administer during CPR? |
What is the optimal ventilation rate during CPR in patients with or without an advanced airway? Is it age dependent? |
What is the optimal chest compression rate during CPR? Is it age dependent? |
What are the optimal blood pressure targets during CPR? Are they age dependent? |
Can echocardiography improve CPR quality or outcomes from cardiac arrest? |
Are there specific situations in which advanced airway placement is beneficial or harmful in OHCA? |
What is the appropriate timing of advanced airway placement in IHCA? |
What is the role of ECPR for patients with OHCA and IHCA due to noncardiac causes? |
What is the optimal timing and dosing of defibrillation for VF/pVT? |
What clinical tools can be used to help in the decision to terminate pediatric IHCA and OHCA resuscitation? |
What is the optimal blood pressure target during the post–cardiac arrest period? |
Should seizure prophylaxis be administered post cardiac arrest? |
Does the treatment of postarrest convulsive and nonconvulsive seizure improve outcomes? |
What are the reliable methods for postarrest prognostication? |
What rehabilitation therapies and follow-up should be provided to improve outcomes post arrest? |
What are the most effective and safe medications for adenosine-refractory SVT? |
What is the appropriate age and setting to transition from (1) neonatal resuscitation protocols to pediatric resuscitation protocols and (2) from pediatric resuscitation protocols to adult resuscitation protocols? |
CPR indicates cardiopulmonary resuscitation; ECMO, extracorporeal membrane oxygenation; ECPR, extracorporeal cardiopulmonary resuscitation; FIO2, fraction of inspired oxygen; IHCA, in-hospital cardiac arrest; IO, intraosseous; IV, intravenous; OHCA, out-of-hospital cardiac arrest; pVT, pulseless ventricular tachycardia; SVT, supraventricular tachycardia; and VF, ventricular fibrillation. |
The American Heart Association requests that this document be cited as follows: Topjian AA, Raymond TT, Atkins D, Chan M, Duff JP, Joyner BL Jr, Lasa JJ, Lavonas EJ, Levy A, Mahgoub M, Meckler GD, Roberts KE, Sutton RM, Schexnayder SM; on behalf of the Pediatric Basic and Advanced Life Support Collaborators. Part 4: pediatric basic and advanced life support: 2020 American Heart Association Guidelines for Cardiopulmonary Resuscitation and Emergency Cardiovascular Care. Circulation. 2020;142(suppl 2):S469–S523. doi: 10.1161/CIR.0000000000000901
- Alexis A. Topjian, MD, MSCE, Chair
- Tia T. Raymond, MD, Vice-Chair
- Dianne Atkins, MD
- Melissa Chan, MD
- Jonathan P. Duff, MD, MEd
- Benny L. Joyner Jr, MD, MPH
- Javier J. Lasa, MD
- Eric J. Lavonas, MD, MS
- Arielle Levy, MD, MEd
- Melissa Mahgoub, PhD
- Garth D. Meckler, MD, MSHS
- Kathryn E. Roberts, MSN, RN
- Robert M. Sutton, MD, MSCE
- Stephen M. Schexnayder, MD
On behalf of the Pediatric Basic and Advanced Life Support Collaborators
The authors thank the following individuals (the Pediatric Basic and Advanced Life Support Collaborators) for their contributions: Ronald A. Bronicki, MD; Allan R. de Caen, MD; Anne Marie Guerguerian, MD, PhD; Kelly D. Kadlec, MD, MEd; Monica E. Kleinman, MD; Lynda J. Knight, MSN, RN; Taylor N. McCormick, MD, MSc; Ryan W. Morgan, MD, MTR; Joan S. Roberts, MD; Barnaby R. Scholefield, MBBS, PhD; Sarah Tabbutt, MD, PhD; Ravi Thiagarajan, MBBS, MPH; Janice Tijssen, MD, MSc; Brian Walsh, PhD, RRT, RRT-NPS; and Arno Zaritsky, MD.
Open table in a new window.
Open table in a new window.